07:30 | Introduction to Microfluidics Training Course. **Separate Registration Required to Attend** Shuichi Takayama, Professor, Georgia Research Alliance Eminent Scholar, and Price Gilbert, Jr. Chair in Regenerative Engineering and Medicine Wallace H. Coulter Department of Biomedical Engineering, Georgia Institute of Technology & Emory University School of Medicine, United States of America
Introduction to Microfluidics Training Course **A Training Course for Beginners and New Entrants into the Microfluidics Field* Date: Wednesday, 29 November 2023 Time: 07:30am-09:00am Location: Ballroom A Coffee and Pastries will be Served This presentation will introduce basics of microfluidics. - Topics include size scales of microfluidic devices and how that affect microscale fluid flows.
- The evolution and different methods of microfluidic device fabrication.
- Select biological applications including cellular, molecular, and exosome applications.
- Some of the challenges and opportunities and future perspectives will also be discussed.
- Time will also be reserved for questions and discussions.
**This is an excellent course for new entrants seeking an immersion into the microfluidics field -- the course is taught by Professor Shu Takayama, a World Leader in the Lab-on-a-Chip and Microfluidics Field.**
**Separate Registration Required to Attend this Course**
|
| Exhibit Hall Opens at 08:00 - Coffee, Tea and Pastries Served in the Exhibit Hall |
| |
| Main Conference Programming Starts at 09:00 |
| |
| Session Title: Deep Dive into Microfluidics and Lab-on-a-Chip Spaces -- Ballroom A |
| |
| Session Chairperson: Dr. Claudia Gärtner, CEO microfluidic ChipShop GmbH Germany |
| |
09:00 | 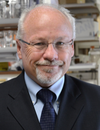 | Keynote Presentation Sequencing Single RNA Molecules using a Nanofluidic Device with Dual In-Plane Nanopore Sensors and Immobilized Exoribonuclease Enzymes Steve Soper, Foundation Distinguished Professor, Director, Center of BioModular Multi-Scale System for Precision Medicine, The University of Kansas, United States of America
With the development of next generation sequencing (NGS), the field of transcriptomics has seen tremendous advancements opening up opportunities for improved diagnostics of diseases such as cancers and infectious diseases. RNA sequencing enables measurement of single nucleotide variants (SNVs), insertions and deletions, detection of different transcript isoforms, splice variants, and chimeric gene fusions. Although NGS has been useful for unraveling RNA structure and function, several technical difficulties remain including the need for reverse transcription and PCR amplification, which can mask epitranscriptomic modifications. To address NGS issues, we are developing an exciting new sequencing technology called Exonuclease Time-of-Flight (X-ToF) for the label-free detection and identification of single molecules. The hypothesis behind X-ToF is, “individual molecules moving electrokinetically through a 2D nanotube will experience a time-of-flight (ToF) that is dependent upon its molecular identity.” X-ToF is a nanofluidic device comprised of input/output channels, a nanoscale solid-phase enzymatic bioreactor, and a flight tube equipped with a pair of in-plane nanopores to measure the ToF of a single ribonucleotide monophosphate (rNMP). Each rNMP is produced from an unamplified RNA molecule being clipped with a processive exoribonuclease, XRN1. In this presentation we will discuss the high rate manufacturing of the X-ToF chip with sub-5 nm in-plane nanopores using nano-injection molding from a cyclic olefin polymer (COP) plastic. The in-plane pores are situated at either end of a nanochannel (50 x 50 nm; 10 µm long) that generates current transient signals to detect and deduce the identity of rNMPs. The ToF is dependent on the apparent electrophoretic mobility of the molecule. The identity is determined from the ToF, the current transient amplitudes, and dwell times using multi-parameter Principle Component Analysis (PCA) or machine learning. We will show the ability to detect (detection efficiency ~100%) single rNMPs with identification accuracies >99% in a single read. Finally, we will discuss the generation of solid-phase nano-bioreactors using XRN1, which can cleave ssRNA in the 5' to 3’ direction releasing single rNMPs that are detected and identified using the in-plane nanopore sensor. Unique properties of the immobilized enzyme will be presented in terms of its processivity and kinetic rate of cleaving single RNA molecules. |
|
09:30 | 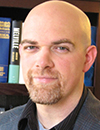 | Keynote Presentation Quantitative Biology with Droplet Microfluidics Adam Abate, Professor of Bioengineering and Therapeutic Sciences, University of California-San Francisco, United States of America
Many questions at the forefront of biology depend on the individual properties and interactions of millions of single cells. My lab develops methods for analyzing, sorting, and engineering single cells using droplet-based microfluidics. I will describe methods in which we are using this to detect rare cells in a population and evolve new cells and enzymes with enhanced properties. |
|
10:00 |  | Keynote Presentation CRISPR-based Diagnostics: Gross Errors, Useful Specificity, and Microfluidic Assays Juan Santiago, Charles Lee Powell Foundation Professor, Stanford University, United States of America
Molecular diagnostics based on clustered regularly interspaced short palindromic repeats (CRISPR) enzyme systems have been the subject of intense research and development. CRISPR-associated (Cas) enzyme assays are easily reconfigurable to different nucleic acid targets, highly specific, and compatible with simple kits and microfluidic components. We are conducting studies of the basic CRISPR enzyme kinetics. We discovered that the great majority of all CRISPR enzyme kinetics studies show data that are inconsistent and which grossly violate basic rules of mass conservation and rate laws. This widespread inconsistency makes it difficult to assess the potential of CRISPR as a diagnostics tool. Following up on this, we quantified the kinetics of a range of CRISPR-Cas systems and demonstrated how these kinetics fundamentally limit detection sensitivity. We also performed a study of CRISPR specificity to small mutations, including single-nucleotide polymorphisms. We are currently developing assays that leverage CRISPR specificity for cancer detection. We will also present a review of microfluidic CRISPR assays and report on our CRISPR assays using on-chip electric field control with a method called isotachophoresis (ITP). |
|
10:30 | Mid-Morning Coffee Break and Networking in the Exhibit Hall |
11:00 | Integration of Mixed Materials Components for Microfluidic Design and Manufacturing Stefano Begolo, Director of Microfluidic Engineering, ALine, Inc.
In order to achieve the desired functionalities, microfluidic products typically require integration of multiple components produced with different materials. Selecting the right integration strategies and number of components is critical to enable scale up manufacturing at target cost. In this presentation we will review examples of components and integration strategies from commercial products, and showcase hybrid glass/plastic devices developed in collaboration by ALine and IMT.
|
11:30 | Consumables for Life Sciences and Diagnostics Made of Glass Tobias Bauert, Business Development Manager, IMT Microtechnologies
Material choice for microfluidic flow cells or biochips is not so much a choice in many cases but a given specification. The application defines the material. Microfluidic consumables made of glass dominate the applications where photonics meet microfluidics. Scaling up from proof-of-concept prototypes to the lab-on-a-chip application in large quantities is full of challenges. One of these challenges is integration of glass microfluidic chips in a system or cartridge. Glass microfluidic chips and sensors can be integrated into plastic cartridges to make use of the best of both worlds: get the high quality and material properties of glass and combine it with an easy to use plastic cartridge.
|
12:00 | Elveflow, Microfluidics One-Stop-Shop: PDMS Microfabrication and Flow Control Théo Champetier, Technical Sales Engineer, Elvesys
Elveflow develops state-of-the-art microfluidics equipment so scientists can focus on the science while we take care of the instruments. We specialize in chip microfabrication in PDMS and high-performance automated flow control, with solid expertise in system design for countless applications. Our plug-and-play microfluidics packs provide easy access to microfluidics for non-specialists.
Joint presentation with Anatole Héliot.
|
12:30 | Networking Buffet Lunch in the Exhibit Hall -- Networking with Colleagues, Engage with Exhibitors, View Posters |
| Session Title: 3D-Printing of Microfluidics |
| |
| Session Chairperson: Professor Albert Folch, University of Washington |
| |
14:00 |  | Keynote Presentation Progress in Advanced 3D Printing for Microfluidics Gregory Nordin, Professor, Brigham Young University, United States of America
While there is great interest in 3D printing for microfluidic device fabrication, a main challenge has been to achieve feature sizes that are in the truly microfluidic regime (<100 µm). A key issue is that microfluidic devices are comprised primarily of negative space features, which therefore dominate 3D printing resolution requirements, as compared to positive space features that are typical for many other 3D printing applications. Consequently, we have developed our own stereolithographic 3D printers and materials that are specifically tailored to meet these needs. We have shown 3D printed channels as small as 18 µm x 20 µm, and have recently reduced this to 2 µm x 2 µm. We have also developed active elements such as valves and pumps. With these capabilities, we demonstrate highly integrated 3D printed microfluidic devices such as a 10-stage 2-fold serial dilutor that simultaneously creates a 3 order of magnitude range of concentrations, high density chip-to-chip interconnects (53 interconnects per square mm) that are directly 3D printed as part of a device chip, and droplet-on-demand structures to efficiently entrain individual bacteria in relatively few droplets despite initial large sample volume. These advances open the door to 3D printing as a replacement for expensive cleanroom fabrication processes, with the additional advantage of fast (~5-15 minute), parallel fabrication of many devices in a single print run due to their small size. |
|
14:30 | DLP 3D Printing: Modern Technology for In-House Manufacturing Ken Wang, Technical Manager, ASIGA
Learn how DLP 3D printing produces accurate, fast, and economical microfluidic devices with Asiga’s proprietary technologies and open material platform.
|
15:00 |  | Keynote Presentation Design Principles For 3D-Printed Microfluidics Noah Malmstadt, Professor, Mork Family Dept. of Chemical Engineering & Materials Science, University of Southern California, United States of America
As 3D printing replaces traditional clean room manufacturing for microfluidic engineering applications, it’s becoming clear that this transition offers not only lower cost and faster design iterations, but also new opportunities for fluidic routing and control that are only possible due to the inherent three-dimensional nature of these systems. Over the past several years, we have developed design principles that take advantage of this three-dimensionality, as well as demonstrating several applications that benefit from this approach.
Designing fluidic paths in three dimensions can be facilitated using standard finite element modeling tools for fluid simulations. For instance, we used a FEM fluid mechanics simulation to design and optimize a 3D flow-focusing junction for manufacturing lipid vaccine nanoparticles. In addition, modular 3D printed devices allow for the application of rules from circuit design. And the nature of 3D printing as an easily characterized manufacturing technique facilitates the statistical analysis of tolerances to predict operational ranges of microfluidic systems.
3D printing also presents materials opportunities and challenges for microfluidic applications. For instance, stereolithographic resins can often poison enzymatic reactions, limiting applications to biochemical processing. We have explored surface modification techniques to passivate 3D-printed channel surfaces and enable on-chip enzymatic reactions. |
|
15:30 | Microfluidics and Direct Laser Write Technology: How to Make the Right Choice? Nicolas Brillouet, CTO, Kloé
Direct laser write technology is really appreciated when considering the fabrication of microchips, and particularly in microfluidics, since it first offers the flexibility of fast prototyping, meaning fabricating at minimum time and development costs, by preventing, in particular, from the need to fabricate some several photomasks before achieving the goals in terms of performance and functionality. Direct laser write technology also enables producing microchips in small and medium series with a very satisfying yield, thanks to the high repeatability of laser processing technology. However, all direct laser writers are not all as suitable as some others to particularly address microfluidics challenges and making the choice of the right equipment/supplier remains very important to ensure that the performance of the equipment is fully compatible with the expected performance/rendering, in particular in terms of edge roughness and verticality, resolution, depth of focus, writing strategy and maximum versatility. This presentation will highlight the most important specifications/parameters that must be considered and thus how to ensure making the right choice of equipment.
|
16:00 | Mid-Afternoon Coffee Break and Networking in the Exhibit Hall |
16:30 | Standardization Meets Customization Claudia Gärtner, CEO, microfluidic ChipShop GmbH
The Shortcut to your Microfluidic Device.
|
17:00 | The Smaller Dimension – The Relevance of Nano-Scale Precision in Clinical Diagnostics Magdalena Schimke, Business Development and Sales, Single Cell Diagnostics, STRATEC Consumables GmbH
Microfluidics (10^-6) is nowadays well established in the world of clinical diagnostics and single cell analysis. Many novel applications however strongly benefit from nano-scaled (10^-9) structural features and precision to allow analysis of rare biomarkers or phenotypical single-cell characteristics. We will discuss examples of both aspects and display the mitigation of challenges in upscaling such high-accuracy consumables.
|
17:30 | Scaling Up PDMS Microfluidic Chips: Prototyping, Production Challenges, and Transition to Injection Molding Jing Chen, CEO, HiComp Microtech
This talk covers the spectrum of PDMS microfluidic chip manufacturing, spanning from initial prototyping to mass production and the transition to plastic injection molding. It begins with an exploration of various prototyping methods and their practical applications, followed by an overview of the demand for volume PDMS chip production and its applications in life science. Challenges in scaling up from prototyping to mass production are examined, encompassing mold issues, consistency, and scalability, along with strategies for overcoming these obstacles. Furthermore, the talk delves into emerging trends in PDMS manufacturing and the potential of PDMS injection molding. A detailed case study illustrates a successful transition from PDMS to injection-molded microfluidic chips, showcasing the benefits of enhanced production efficiency and reduced costs.
|
18:00 |  | Keynote Presentation Microfluidic T Cell Engineering For Immunotherapies Abraham Lee, Chancellor’s Professor, Biomedical Engineering & Director, Center for Advanced Design & Manufacturing of Integrated Microfluidics, University of California-Irvine, United States of America
Adoptive cell therapy (ACT) is a type of immunotherapy that involves the processing of blood from a donor to isolate immune cells (e.g. T cells) for genetic manipulation followed by reinfusion of the cells into patients. Specifically for CAR T cell therapy, genetic coding material (e.g. DNA, mRNA) is inserted into the T cells to express chimeric antigen receptors to target biomarkers of cancer cells and trigger an activated immune response towards the tumor of interest. This process that starts from blood drawn from one person and ends with specialized engineered cells delivered to the same patient includes multiple tedious and costly steps, and can require a long time that the patient may not have. Microfluidics techniques are being developed that can address all steps of this cell manufacturing process, including cell harvesting, cell isolation, cell activation and expansion, and cell transfection. In this talk I will introduce two microfluidic platforms in my lab, one is the lateral cavity acoustic transducer (LCAT) and the other is droplet microfluidics. LCAT was used for processing blood samples, isolating T cells, transfecting T cells, and finally expanding T cells to scale up for treatment. Based on LCAT, we developed the acoustic electric shear orbiting poration (AESOP) device to uniformly deliver genetic cargo dosage into a large population of cells simultaneously. Based on droplet microfluidics we constructed a single cell artificial antigen presenting cells (aAPCs) for T cell activation. By trapping single cells in microfluidic compartments, we are able to study the cell morphology and cell-cell communications to further understand immune cell activation and immune cell synapses. |
|
18:30 | Networking Reception with Beer and Wine in the Exhibit Hall - Engage with Exhibitors and View Posters |
19:30 | Close of Day 2 Conference Programming |