Monday, 9 February 201508:00 | Conference Registration, Coffee, and Breakfast Pastries | 08:50 | Chairman's Opening Remarks Jon Rowley, Chief Executive And Technology Officer, Rooster Bio Inc, United States of America
| | Session Title: Emerging Themes and Research Trends in Bioprinting |
| | | Session Chairman: Jon Rowley, Ph.D., CEO, RoosterBio |
| | 09:00 | 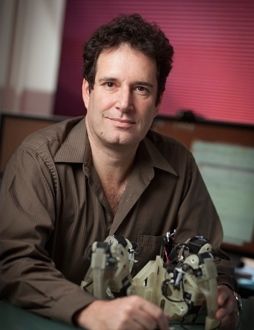 | Keynote Presentation The Next 25 Years of 3D Printing Hod Lipson, Professor, Cornell University, United States of America
Additive manufacturing has evolved over the last three decades from limited and expensive prototyping equipment in the hands of few, to commodity production tools available to almost anyone. It’s been broadly recognized that this burgeoning industrial revolution will transform almost every industry, and every aspect of our lives. But where will this technology go next? This talk will describe the underlying disruptive future of 3D printing: From printing arbitrarily complex shapes to creating new kinds of materials, and ultimately, moving from fabricating passive parts to printing active, integrated systems, including electronics, actuators and sensors. Will we be able to print a robot that will walk out the printer, batteries included? |
| 09:45 | 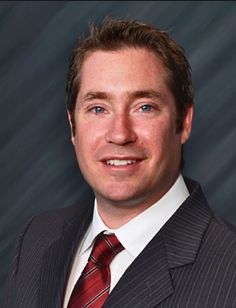 | Keynote Presentation Commercial Opportunities for Bioprinting Keith Murphy, CEO, Organovo, United States of America
Bioprinting is opening up new technical solutions for many disciplines. Academic and industrial scientists are working to expand the technologies and the uses of bioprinting. The commercial opportunities are broad, and are still being explored. There are many hurdles to commercial maturity, but the key to success is the technologies in the field, which are already in fast-paced development and will ultimately lead the way. |
| 10:30 | Coffee Break, Networking with Exhibitors, and Poster Viewing | 11:00 | 3D Bioprinting of Vascularized Living Tissue Jennifer Lewis, Professor, Harvard University School of Engineering and Applied Sciences, United States of America
The ability to pattern biomaterials in planar and three-dimensional forms is of critical importance for several applications, including 3D cell culture, tissue engineering, and organ mimics. 3D printing enables one to rapidly design and fabricate soft materials in arbitrary patterns without the need for expensive tooling, dies, or lithographic masks. In this talk, the design of novel cell-laden, hydrogel (extracellular matrix) and fugitive (vascular) inks with tailored rheological properties for 3D bioprinting will be described. We will also present recent advances in 3D bioprinting of vascularized, heterogeneous cell-laden tissue constructs with as well as ongoing efforts to characterize these 3D living architectures. | 11:30 | 3D Biofabrication of Complex Living Cardiovascular Tissues: Past, Present, Future Jonathan Butcher, Associate Professor, Biomedical Engineering, Cornell University, United States of America
Cardiovascular disease remains a leading cause of death worldwide. Despite their potential, achieving functional living tissue replacements has been an elusive “Holy Grail” for the last two decades. Among the challenges is that natural tissues are highly complex, hierarchical structures that are difficult to replicate. Tissue biofabrication technology, in particular 3D tissue printing, has made significant strides over the past 5 years to close this gap. 3D tissue printing has the capacity to prescribe both macro and microstructural environmental cues that are essential for coordinating whole tissue function. We discuss recent findings on two prominent applications: trileaflet heart valves and vascularized tissue flaps. | 12:00 | 3D BioFabrication for Biological Machines and Tissue Engineering Rashid Bashir, Professor And Head, University Of Illinois, United States of America
The integration of living cells with soft scaffolds can enable the fabrication of biological machines and soft robotics. These cell-based biological machines can be defined as a set of sub-components consisting of living cells and cell-instructive micro-environments that could eventually perform a range of prescribed tasks. The realization of biological machines and their sub-components will require a number of suitable cell sources, biomaterials, and enabling technologies. Here, we review our group’s recent efforts towards this goal and of developing cell based biological machines. We have fabricated locomotive ‘‘bio-bots’’ from hydrogels and cardiomyocytes using a 3D printer. The multi-material bio-bot consisted of a ‘biological bimorph’ cantilever structure as the actuator to power the bio-bot, and a base structure to define the asymmetric shape for locomotion. The cantilever structure was seeded with a sheet of contractile cardiomyocytes. We will also describe the development of a 3D-printed electrically paced skeletal muscle based ‘bio-bot’ devices where skeletal myoblasts embedded in ECM proteins compacted around a hydrogel structure were used to create the power source of the biological walking machine. While the specific applications are yet to be defined, these devices could have potential applications in drug delivery, power generation, and other biomimetic systems. | 12:30 | Lunch, Networking, Poster Viewing and Discussion Round Tables | | Session Title: Technologies and Engineering Methodologies for Bioprinting |
| | | Session Chairman: Hod Lipson, Ph.D., Professor, Cornell University |
| | 13:30 | Tools for the Development of Bioprinting Technology Jeffrey Lipton, Founder & Chief Technology Officer, SERAPH ROBOTICS, United States of America
3D bio printing is a field with unique technical needs and requirements in the 3D printing community. Bio printing, being in the early stages of process development, needs flexible platforms to allow rapid innovation. Current 3D printer architectures are ether locked down and inaccessible to researchers, or have a high cost and technical barrier. We present the XDFL and Fab@Home printer frameworks as an alternative to these barriers to innovation. | 14:00 | 3D Scaffolds with Tailorable Topology and Topography through Biofabrication Technologies Lorenzo Moroni, Professor, Biofabrication for Regenerative Medicine, Maastricht University and Founder MERLN Institute for Technology-Inspired Regenerative Medicine, Netherlands
A key factor in scaffold-based tissue and organ regeneration relies on enhancing (stem) cell-material interactions to obtain the same original functionality. Different approaches include delivery of biological factors and surface topography modifications. Although both strategies have proved to augment cell activity on biomaterials, they are still characterized by limited control in space and time, which hampers the proper regeneration of complex tissues. Here, we present a few examples where the integration of biofabrication technology platforms allowed the generation of a new library of 3D scaffolds with tailored biological, physical, and chemical cues at the macro, micro, and nano scale. By engineering their topological properties, these porous biomaterials influence the activity of seeded cells, thereby initiating the regeneration of skeletal, vascular, and neural tissues. Future efforts should aim at further improving our understanding of scaffold topological properties to achieve a fine control on cell fate at multiple scales. This will enable the regeneration of complex tissues including vasculature and innervation, which will result in enhanced in vivo integration with surrounding tissues. By doing so, the gap from tissue to organ regeneration will be reduced, bringing regenerative medicine technologies closer to the clinics. | 14:30 | Extrusion Printing of Hydrogels with Embedded Cells Paul Calvert, Professor of Bioengineering, UMass Dartmouth, United States of America
A 3D printer has been used to make porous open gel “logpiles” with 200 micron bars containing embedded cells including yeast, fibroblasts and algae. The activity of the cells has been studied as a function of gel composition. As expected, cells can metabolize and multiply within a 100 microns of the gel surface. Following the activity of a yeast membrane-bound invertase and comparing this with known diffusion coefficients allows us to quantitatively model metabolism. .The gel formulations need to give good strength coupled with easy diffusion of nutrients and products will be discussed. Diffusion coefficients have also been measured for a number of macromolecules in different types of printed gels in order to determine the possibility of using encapsulated cells in 3D gel scaffolds as small bioreactors to produce proteins. | 15:00 | Coffee Break, Networking, Exhibit and Poster Viewing | 15:30 | Melt Electrospinning in a Direct Writing Mode – A New Additive Manufacturing Technology Platform Dietmar Hutmacher, Professor, Queensland University of Technology, Australia
It has long been appreciated that the electrostatic drawing (electrospinning) of either polymer solutions or melts is possible. Research into melt electrospinning, however, is significantly less and comparatively recent - with over half of the melt electrospinning literature being published since 2011. Even with this recent increase in number of publications, melt electrospinning contributes to less than 1% of the solution electrospinning literature. There is compelling rationale for electrospinning fibres from the melt in certain applications. The use of solvents can be problematic, and extraction during electrospinning and post-processing are undesired. This is particularly true in biomedical materials applications, where the volatile solvents used in solution electrospinning are also toxic to cells and tissues. There are also important phenomenological aspects of electrospinning polymer melts, and recent research applying additive manufacturing principles to the process has resulted in new structures for tissue engineering applications. | 16:00 | Development of Bioinks for 3D-Bioprinting Jos Malda, Professor of Biofabrication in Translational Regenerative Medicine, University Medical Centre Utrecht, Netherlands
Hydrogels are particularly attractive as “bioinks” for biofabrication as they recapitulate several features of the natural extracellular matrix and allow cell encapsulation in a highly hydrated mechanically supportive 3D environment. Additionally, they allow for efficient and homogeneous cell seeding, can provide biologically-relevant chemical and physical signals and can be formed in various shapes and biomechanical characteristics. Nevertheless, there exists a significant challenge in biofabrication: the optimization of – intrinsically weak – hydrogels to address the physico-chemical demands of the biofabrication process and the right conditions for cell survival on the one hand, and to address the harsh in vivo mechanical environment on the other. We have developed novel hydrogel-based bioink formulations that allow for the construction of intricate 3D structures, whilst providing the cells with a biologically suitable environment. | 16:30 | Bioprinting and Bioassembly of Bioficial Organs Stuart Williams, Director, Bioficial Organs, University of Louisville, United States of America
The assembly of functional organs from a patient’s own tissue and cells remains a major goal of regenerative medicine. With the development of computer assisted design of tissues and organs and the integration of additive manufacturing technologies the era of 3D Bioprinting has emerged as a new technology toward the manufacturing of custom organs. This presentation will describe the next generation of bioprinting systems that include new multi-axis robotic systems. Advancements in biomaterials have created new “bioinks” a critical component of tissue and organ 3D bioprinting. The field of 3D Bioprinting is positioned to become a major clinical tool to address numerous diseases as well as aid in the development of new diagnostic tests and in drug development. | 17:00 | Applications Development with 3D Human Tissues created via Additive Manufacturing Approaches Sharon Presnell, Executive Vice President, R&D , Organovo, Inc., United States of America
Additive manufacturing is emerging as a compelling means to generate three-dimensional structures comprising living cells for use in in vitro and in vivo applications, with an exceptional degree of architectural control. The value of tissues produced using these methodologies will be determined, ultimately, by their functional performance in in vitro and in vivo applications. Phenotypic and functional attributes of 3D-bioprinted human tissues, such as liver, will be discussed. | 17:30 | High Performance Additive Manufacturing for Long-Term Implantable Devices Adam Hacking, Chief Scientific Officer, Oxford Performance Materials (OPM), United States of America
High performance additive manufacturing (HPAM) describes the direct fabrication of fully functional, mission critical devices. HPAM of medical devices requires an understanding of material properties, manufacturing techniques, device design and biological performance. Oxford performance materials has developed a unique laser sintering process with a high performance thermoplastic, poly-ether-ketone-ketone (PEKK) that enables the generation of load-bearing additively manufactured devices. OPM is the first company to have obtained FDA clearance for additively manufactured polymeric devices for long-term implantation. This talk will introduce OPM's technology, describe the development and commercialization process, provide an overview of the biological response to PEKK devices and outline current development. | 18:00 | 4D Bioprinting: A New Paradigm for Engineering Complex Tissues Fabien Guillemot, CEO, Poietis, France
Dealing with tissue complexity and reproducing the functional anisotropy of human tissues remain a puzzling challenge for tissue engineers. Emergence of the biological functions results from dynamic interactions between cells, and with extracellular matrix. The important literature showing that cell fate (migration, polarization, proliferation…) is triggered by biochemical and mechanical signals arising from cell microenvironment suggests that tissue formation obeys to short range orders without reference to a global pattern. In that context, the winning tissue engineering strategy might rely on controlling tissue organization at the cell level. Emerging during the last decade, Bioprinting has been defined as “the use of computer-aided transfer processes for patterning and assembling living and non-living materials with a prescribed 2D or 3D organization in order to produce bio-engineered structures serving in regenerative medicine, pharmacokinetic and basic cell biology studies”. From a technological point of view, the Laser-Assisted Bioprinting (LAB) technology has been developped as an alternative method to inkjet and bioextrusion methods, thereby overcoming some of their limitations (namely clogging of print heads or capillaries) to pattern living cells and biomaterials with a micron-scale resolution. By harnessing this high printing resolution, we observe that tissue self-organization over time depends on the cell patterns initially printed by LAB, as well as cell types. To engineer complex tissues, we then emphasize the need to consider the spatio-temporal dynamics of tissue self-organization when designing blueprints. | 18:30 | Networking Reception: Enjoy Premium Beers, California Wines, and Appetizers with Your Colleagues with a Beautiful View of Boston and The Charles River | 20:00 | Close of Day 1 of the Conference |
Tuesday, 10 February 201508:00 | Morning Coffee and Breakfast Pastries | | Session Title: The Current Status of Tissue Engineering and its Impact in the Clinic |
| | | Session Chairman: Oren Levy, Ph.D., Brigham and Women's Hospital/Harvard Medical School |
| | 08:30 | Why Manufacturing Matters: How Today’s Cell Therapy BioProcess Innovations are Laying the Foundation for a Sustainable Tissue Engineering Revolution Jon Rowley, Chief Executive And Technology Officer, Rooster Bio Inc, United States of America
Technology is rapidly moving toward the integration of biologics into products like cell therapies, engineered tissues, bio-robotics, implantable devices, 3D printing, food, clothing, and even toys. This coming decade will see the incorporation of living cells into all these platforms and others not yet imagined. To expedite this biologics revolution, inventors, developers and suppliers will require a limitless, standardized, low-cost supply of living cells – and today’s cell therapy bio-manufacturing innovations are laying the groundwork to make this a reality. | 09:00 | Building Blocks for Tissue Engineering and Disease Models in 2D and 3D Mark DeCoster, Associate Professor, Louisiana Tech University, United States of America
In tissue engineering, bioprinting, and biofabrication, we must consider cell-cell contacts, and cell-materials contacts. These are the building blocks that start at 2 dimensions (2D) and then are built up into 3 dimensions (3D). This presentation will discuss some of our work utilizing 2D and 3D systems for tissue engineering and for disease models, including building blocks of 3D cell spheroids, 3D printed materials, and materials of different shapes and sizes for biofabrication, including magnetically susceptible materials and materials treated to release drugs. As a neuroscience lab, we are interested in how the different cells of the brain communicate with each other, both as normal cells, and in disease states such as cancer (brain tumor cells). Tissue engineering and biofabrication techniques are already improving our knowledge of understanding how to work with normal cells in 3D and providing more relevant 3D models for diseases such as cancer. One theme of the presentation will include considerations of the dynamics of cells—for example, cells at a 2D/3D interface may not be morphologically or functionally identical to those surrounded only by other cells. It is anticipated that these interfaces will be key in understanding tissue engineering and bioprinting that is 3-dimensional and anisotropic. | 09:30 | 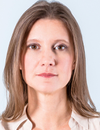 | Keynote Presentation Organs-on-Chips: Highly Functional Microphysiological Systems to Predict Human Physiology and Pathobiology Geraldine A Hamilton, President/Chief Scientific Officer, Emulate Inc, United States of America
This presentation will review our novel biomimetic microsystem technologies and discuss their potential application in predicting efficacy, safety and mechanism of action for new drugs, chemicals, and cosmetics. Biomimetic Organs-on-Chips offer exciting new approaches to attack fundamental questions in biology, and develop smart in vitro surrogates for regulatory sciences that can positively impact human health. We apply microfabrication approaches to engineer cell culture microenvironments that go beyond conventional three-dimensional cell culture models by recapitulating the tissue-tissue interfaces, spatiotemporal chemical gradients, mechanical microenvironments, and physiological function of living organs. These Organs-on-Chips are being combined with cultured human cells to study human physiology in an organ-specific context. The presentation will also highlight the potential application of these systems for disease model development, standing as more predictive, human-relevant alternatives to current animal-based models. |
| 10:15 | Coffee Break, Networking, Exhibit and Poster Viewing | 10:45 | Using Microfluidics to Grow Perfusable Vascular Networks Roger Kamm, Cecil and Ida Green Distinguished Professor of Biological and Mechanical Engineering, Massachusetts Institute of Technology (MIT), United States of America
For years, one of the major hurdles in the creation of engineered tissues was the unmet need for a microvascular network. Recently, several groups have reported the development of microfluidic systems incorporating 3D co-culture of endothelial cells and fibroblasts to produce perfusable networks of microvessels, paving the way for more complex systems with other cell types for specific organ or tissue functions. These in vitro systems can be maintained long-term, raising the prospect of generating complete tissues in vitro for various purposes including organs for implantation or non-medical applications. Several challenges yet need to be addressed, including the control of network morphology and stabilization of the networks once established. Both the current state-of-the-art and the directions of future research will be addressed. | 11:15 | Microfluidic Microvasculature for Tissue Engineering Rong Fan, Harold Hodgkinson Professor of Biomedical Engineering, Yale University, United States of America
A major challenge in tissue engineered organ transplantation is revascularization. How to fabricate a perfusable microvascular network in neotissue to support the tissue growth in vivo is crucial. We are working to address this problem by developing a two-step approach for synthesizing neotissues with perfusable microvasculature. First we use a microfluidic system to create a large-scale endothelialized microvessels that can be retrieved to form a free-standing microvascular network. Second, this microvascular network is used as a template to seed perivascular and tissue specific cells to grow neotissues. This modular approach is generic and versatile for the potential application to a range of functional tissues including liver, bone, and pancreatic tissues. | 11:45 | Engineering Vascularized Craniofacial Bone Grafts Warren Grayson, Assistant Professor, Johns Hopkins University School of Medicine, United States of America
The treatment of large craniofacial bone loss due to congenital defects, trauma or cancer resection remains a huge clinical challenge. There are approximately one million fractures requiring bone transplantation annually in the US and it is predicted that within the next 10 years this number will significantly increase, particularly in persons over 50 years of age. Tissue engineering provides a viable means of obtaining ‘autologous’ bone grafts for the treatment of large bone defects. Successful application of tissue-engineered grafts however requires that we can couple the formation of de novo vasculature in tandem with new bone growth. Our lab has developed techniques for 3D printing anatomically-shaped bone scaffolds and pre-vascularizing these scaffolds using adipose-derived stem cells (ASCs) to engineer vascularized bone grafts that can be used to repair craniofacial defects. | 12:15 | Networking Lunch and Exhibit, Poster Viewing | | Session Title: Tissue Engineering and Bioprinting -- From Research to Commercialization and Impact in the Clinic |
| | | Session Chairs: Geraldine Hamilton, Ph.D., President & CSO, Emulate, and Jon Rowley, Ph.D., CEO, RoosterBio |
| | 13:30 | Bioprinting – The Patent Landscape Robert Esmond, Director, Sterne, Kessler, Goldstein & Fox P.L.L.C, United States of America
Ways to protect bioprinting innovations and patent filings to date will be discussed. A patent landscape search has revealed that there have been many patent filings on bioprinted organs and tissues and methods of production over the last 10+ years. Avoiding patent infringement while in the clinic will be discussed as well as the patent expiration dates. | 14:00 | Bioengineering Strategies for Improved Clinical Impact of Cell-based Therapies Oren Levy, Instructor of Medicine, Harvard Medical School/Brigham and Women’s Hospital, United States of America
A key focus of bioengineering research is to develop effective stem cell therapies to treat a wide range of diseases. This talk will focus on bioengineering strategies to enhance control over cell fate following transplantation. Specifically, approaches to control cell targeting to sites of disease to maximize therapeutic impact and new perspectives in the area of cell therapy will be discussed. | 14:30 | Engineering Synthetic Tissues for Treatment of Chronic Liver Disease Kelly Stevens, Research Scientist, Massachusetts Institute of Technology, United States of America
Cell-based therapies for organ regeneration have recently emerged as a potential alternative to whole-organ transplantation. Unfortunately, orthotopic cell-based therapy may not be feasible or effective in all diseased organs. For example, in end-stage liver disease, the inhospitable fibrotic microenvironment in cirrhotic liver is likely to limit cellular engraftment. Our goal is to build functionally stabilized engineered tissues that can be implanted ectopically and ultimately used to contribute to host liver functions. To create functionally stable engineered liver tissue, we have developed versatile microtissue molding and bioprinting-based methods that enables rapid, scalable, and multicompartmental cellular placement in various patterns and material systems across tissue sizes relevant for in vitro, pre-clinical, and clinical biologic studies. We have used these methods to identify multicellular architectural tissue configurations that best support parenchymal primary human or induced pluripotent stem cell (iPS)-derived hepatocyte survival and function in vitro and in vivo. Ongoing work seeks to extend these findings for application liver repair and regeneration using canonical liver injury model systems. | 15:00 | Coffee Break, Networking, Exhibit and Poster Viewing | 15:30 | Vascular Patches for Pediatric Vascular Tissue Engineering Joyce Wong, Professor of Biomedical Engineering and Materials Science & Engineering, Boston University, United States of America
This talk will focus on methods to generate vascular patches for pediatric vascular tissue engineering applications. We have developed methods involving cell sheet engineering to control the structural organization and functional properties of vascular patches. We will also discuss how computational methods can be integrated with experimental techniques for iterative tissue engineering design and validation. | 16:00 | Three Dimensional Printing of Biodegradable Cardiovascular Biomaterials John Fisher, Fischell Family Distinguished Professor & Department Chair; Director, NIH Center for Engineering Complex Tissues, University of Maryland, United States of America
Rapid advances in three dimensional (3D) printing, whether based on extrusion or lithography, are bringing the technology closer to clinical use in tissue engineering. 3D printing allows for precise bulk geometry and interior architecture control. Based upon material selection, the resolution of the interior and bulk geometry can typically range from 25 µm to 100 µm. Using 3D printing, we have developed biodegradable scaffolds with custom pore sizes. These scaffolds have been non-destructively analyzed with microcomputed tomography to calculate changes in scaffold pore size, porosity, and wall thickness during a 16 week degradation study. One potential clinical application of 3D printing is for the treatment of cardiovascular diseases, through which outcomes may be drastically improved with custom fabrication of patient-specific grafts. Current clinical strategies rely upon surgeons constructing tailor-made implants during surgery with generic grafts. Advancements in imaging technologies, such as MRI and CT, allow for the production of high-quality 3D images from which patient-specific grafts and implants can be generated prior to surgery. To this end, we have formulated a poly(propylene fumarate) (PPF) based resin to 3D print vascular features with mechanical properties similar to native blood vessels. The grafts support the growth of vascular cells in vitro and support good neotissue formation after one and three months in vivo. Further, these grafts may be modified with biomolecules, such as VEGF, so as to recruit and mobilize endothelial cell populations and thus quicken graft endothelialization. | 16:30 | Novel, Cellular Biomarkers Indicating Tissue-specific, Regenerative Potential Eric Darling, Associate Professor of Medical Science, Engineering, and Orthopaedics, Brown University, United States of America
Mesenchymal stem/stromal cells (MSCs) have garnered intense interest for their application in tissue engineering and regenerative medicine therapies. Unfortunately, the heterogeneity inherent in these cell populations complicates their use. Traditional, surface marker-based approaches have had limited success purifying autologous MSCs at sufficient cell yields such that ex vivo expansion is not required. Recently, our group has shown that both single-cell mechanical properties and live-cell gene expression signals can be used to predict the differentiation potential of MSCs. These approaches target all cells in stem/stromal populations that are capable of producing lineage-specific metabolites, encompassing a broader swathe of cell types and differentiation states than traditional techniques. In mechanical property-based experiments, we have shown that less compliant MSCs are more likely to deposit large amounts of calcified matrix compared to more compliant MSCs following osteogenic induction. Conversely, more compliant MSCs showed a propensity to produce large amounts of intracellular lipids following adipogenic induction. In gene expression-based experiments, we have shown that MSCs can be sorted using a fluorescent marker that binds to early osteogenic mRNA molecules, resulting in cell populations that deposited larger amounts of calcified matrix deposition over unsorted controls. Cell yields were also significantly higher than standard, cell enrichment approaches. While osteogenesis has been the primary target of investigation, continuing work is applying these techniques to other cell types and tissues. | 17:00 | The Use Of PRP and Stem Cell injections in an Office Setting Joseph Purita, Medical Director of Stem Cell Centers of America, Institute Of Regenerative and Molecular Orthopedics, United States of America
The presentation concerns PRP and Stem Cell (both bone marrow and adipose) injections for musculoskeletal conditions in an office setting. Indications are given as to which type of cell and technique to use to accomplish repair. Stem cells, both bone marrow derived (BMAC) and adipose, are used for the more difficult problems.. PRP injections are utilized for the less severe tendon problems. Discussed are the indications of when to use Stem Cells verses PRP and when to use both. The newest concepts in stem cell science are presented. These concepts include the clinical use of MUSE cells, exosomes, and Blastomere like stem cells. Basic science of both PRP and stem cells are discussed. This presentation defines what constitutes an effective PRP preparation. Myths concerning stem cells are dispelled. One myth is that mesenchymal stem cells are the most important stem cell. This was the initial interpretation of Dr. Arnold Caplan the father of mesenchymal stem cell science. Dr. Caplan now feels that MSCs have an immunomodulation capacity which may have a more profound and immediate effect on joint chemistry and biology. We learn that the hematopoietic stem cells are the drivers of tissue regeneration. Also discussed are adjuncts used which enhance the results. Therapies include supplements, LED therapy, laser, electrical stimulation, and cytokine therapy. The scientific rationale is presented for each of these entities as to how they have a direct on stem cells. | 17:30 | Human Microphysiological Systems of Blood Vessels and Skeletal Muscle for Drug Toxicity George Truskey, R. Eugene and Susie E. Goodson Professor of Biomedical Engineering, Duke University, United States of America
Skeletal muscle is important for drug and toxicity testing given the relative size of the muscle mass and cardiac output that passes through muscle beds, the key role of muscle in energy substrate metabolism and diabetes, its role in mediating the severity of peripheral arterial disease and heart failure, and the need for therapies for muscle diseases such as muscular dystrophy and sarcopenia. To develop a system for functional and drug testing under physiological conditions, we developed three-dimensional skeletal muscle cultures and tissue engineered blood vessels (TEBV) with a functional endothelial layer. TEBVs are of arteriolar dimensions (inner dimaeters between 400 µm and 800 µm) and vasoconstriction induced by 1 µM phenylephrine was stable over 5 weeks of culture. TEBVs relaxed in the presence of acetylcholine only when endothelial cells were present, consistent with their role in vascular function. The TEBV exhibited responses to inflammatory stimuli suggesting injury and repair. Human engineered muscle bundles exhibited contraction after electrical stimulation and tetanus at high frequency of stimulation. HuMB routinely achieve twitch and tetanic contractile forces > 0.5 mN and1 mN, respectively and exhibit typical Frank-Starling like twitch force-length relationship and passive tension-length relationship. Both TEBV and HuMB exhibited responses to Drugs similar to those observed in vivo. Supported by UH2/UH3TR000505 and the NIH Common Fund for the Microphysiological Systems Initiative. | 18:00 | Sports Medicine and Stem Cells: A Clinical Transformation Dennis Lox, Physician, Florida Spine and Sports Medicine Center, United States of America
Athletic endeavor has always intertwined with pursuing optimal physical performance. This, coupled with the inherent risk of traumatic injury has placed sports medicine at the forefront of progressive treatment. The emergence of Regenerative Medicine has led to the clinical translation of sports medicine related problems. The use of Platelet Rich Plasma (PRP) and Stem Therapy are cornerstones of this model. The scientific literature will be explored to provide a foundation for the pathophysiology of injury and trauma as it relates to sports medicine, and the regulation of catabolic responses through cellular signaling and cytokines. The rationale for the use of Stem Cell Therapy and PRP in sports medicine is presented. Various clinical cases are presented to illustrate the utilization of stem cell therapy and PRP as a therapeutic strategy to assist athletes in their return to sport. Select situations in which standard medical treatment is a surgical intervention, may be unsuitable for return to sport. A Regenerative Medicine treatment model incorporating Stem Cell Therapy, may provide suitable alternative strategies that facilitate healing and repair, without precluding return to sport. In the sports medicine world success is measured by return to sport. The clinical translation of Stem Cell Therapy into sports medicine, may foster a transformation of treatment strategy. | 18:30 | Title to be Confirmed. Gabor Forgacs, Professor, University of Missouri-Columbia; Scientific Founder, Organovo; CSO, Modern Meadow, United States of America
| 19:00 | Close of Day 2 of the Conference |
|